
Image via Wikipedia

Image via Wikipedia
Outline
Change in the planetary winds (conceptually documented in the diagram above) is the least remarked but most influential dynamic affecting surface temperature. Wind is a response to pressure differentials. So, a change in the wind is due to a change in these pressure differentials.
The following post describes why pressure differentials and the the planetary winds change over time.
From Wikipedia we have: “the troposphere is the lowest portion of Earth’s atmosphere. It contains approximately 80% of the atmosphere’s mass and 99% of its water vapor and aerosols. The average depth of the troposphere is approximately 17 km (11 mi) in the middle latitudes. It is deeper in the tropical regions, up to 20 km (12 mi), and shallower near the poles, at 7 km (4.3 mi) in summer, and indistinct in winter.”
The notion that there is a tropopause in high latitudes or that it is somehow ‘indistinct in winter’ represents sloppy thinking. At high latitudes, in winter, the air is not heated by the surface (very cold) or the release of latent heat (a cold desert). Neither is it heated directly by the sun (below the horizon). It is heated by the absorption of long wave radiation from the Earth by ozone. In consequence parts of the polar stratosphere and the troposphere are permanently locked together in convection. Consequently ozone descends into the near surface atmosphere. This process changes the distribution of atmospheric mass and therefore surface pressure. It governs the strength of the planetary winds and cloud cover in the troposphere. The process manifests as the Northern Annular Mode (or the Arctic Oscillation) and the Southern Annular Mode. These well recognized modes of inter-annual climate variation affect mid and high latitude temperatures and winter snow cover. But this is not the half of it. The influence of the circulation at the winter pole extends to the equator and the alternate hemisphere, especially in the case of the Arctic where stratospheric ozone concentration is more elevated than over Antarctica. If we imagine that this phenomenon is responsible for just the inter-annual climate variation, we reveal a blindness to the evolutionary nature of the phenomenon and its capacity to change climate over decadal and longer time scales.
The key to the evolution of surface pressure and wind is the polar night jet that introduces NOx from the mesosphere eroding stratospheric ozone. It is most active in the winter hemisphere when coincidentally, the diminished loss of ozone by photolysis allows ozone levels to increase. But it extends into the spring and autumn and in the case of Antarctica is always active. Spatial variation in ozone concentration determines the pattern of ascent and descent. Convection at high latitudes involves the descent of ozone into the ‘troposphere’ where it moves equator-wards dramatically affects surface pressure, atmospheric relative humidity and therefore the process of condensation that determines cloud cover, the most influential aspect of the Earth’s albedo.
It is observed here: http://www.bishop-hill.net/blog/2011/11/4/australian-temperatures.html that it is temperature change in first half of day that is characteristic of recent climate change. It appears that almost all the warming over the last 60 years in Australia occurred between 6 am and 12 noon. Warming at this time is strongly correlated with decreasing cloud cover during the daytime and is therefore caused by increased solar insolation. Accordingly, we can observe that recent climate change proceeded from change in the Earths atmospheric albedo.
The ‘workings’ of the atmosphere at the poles is a frontier for climate science. See for instance http://www.physics.otago.ac.nz/space/2008JA014029-pip.pdf or search on the words ‘geomagnetic activity Arctic Oscillation Index’ (without the inverted commas).
This essay offers an interpretation of the working of the high latitude atmosphere from the broadest perspective. It takes time for unfamiliar ideas to gain traction but in truth much of what is described here is evident in the literature and is very much a part of the language of meteorology. This is not new knowledge. However, its importance is lost on those who wish to see man as the agent of climate change. This represents a triumph of ideology over observation, an everyday occurrence in the affairs of man.
If you believe that surface temperature depends upon trace gas composition via back radiation it would be best to put that idea aside while you read this paper. Observation of the manner in which the climate at the surface of the Earth changes over time suggests that the effect of down-welling radiation is swamped by the mechanism described here. Historically the globe has warmed and cooled hemispheric-ally rather than monolithic-ally, a point that is lost on those who insist on a single global metric for temperature. The process is hemispheric-ally distinctive because it is driven at the poles. The atmosphere at the two poles is distinctively different, largely due to the very different distribution of land and sea between the hemispheres. It is the difference in the atmosphere between the poles that is important for the evolution of climate.
The Tropical Atmosphere
Viewed from space the troposphere is so thin as to be indistinguishable from the actual surface of the Earth. The Earth has a diameter of 12,756 km. If the Earth were a mattress with a thickness of 300 mm and it were to be covered in a blanket in strict proportion that the troposphere bears to the diameter of the Earth, that blanket would be just 0.35 mm in thickness, the equivalent of five sheets of newspaper. It is the nature of the troposphere that it is hopelessly unstable. Imagine sleeping under five sheets of newspaper with the lowest sheet removed every few seconds and replaced on top. Occasionally someone comes with a watering can to make sure that you are not overheating. The source of radiant warmth from above is lost for a variable portion of a twenty four hour cycle. Unless there is a substantial body of relatively warm water nearby, the surface of the Earth/mattress must soon get very cold. Fortunately, bodies of water have an enormous capacity to store energy and the tropical ocean is a storage organ for the Earth as a whole.
The troposphere efficiently transports energy away from the surface of the Earth via conduction, evaporation and convection. It also transfers energy laterally. But ultimately the surface of the Earth is not warmed by the troposphere, it is warmed by the ocean that traps energy from the sun. Local temperature is primarily dependent upon the place of origin of the wind that is blowing, and whether it is from the land or the sea. The troposphere prevents the surface from overheating. So, surface air temperature is much influenced by the presence or absence of bodies of water and the extent of the sky that is cloudy.
Figure 1 The temperature of the tropical atmosphere at 10°north to 10° south in 2010
Source http://www.cpc.ncep.noaa.gov/products/stratosphere/strat-trop/
From the surface, temperature declines with altitude to the 100hPa pressure level at about 15km. In figure 1 the thermal tropopause, the point at which there is sufficient ozone to reverse the decline of temperature with altitude, is marked with red ovals. Above this point temperature increases with altitude to the stratopause at 1hPa, an elevation of 45km where the temperature can be similar to that at the surface of the Earth. In the mesosphere the temperature of the air falls away with falling ozone concentration. The diagram at upper-right is to scale but there is an error. It shows cloud in the stratosphere. In fact cloud is largely confined to the troposphere because air is de-humidified as it cools during ascent.
At 15km in elevation the tropical tropopause has a temperature of minus 80°C. There is nowhere in the lower atmosphere (stratosphere and troposphere) where the air is colder. Even in the polar night the temperature of the air is greater than minus 80°C. The coolness of the air at the tropical tropopause is a reflection of ozone scarcity. Ozone absorbs long wave radiation from the Earth warming adjacent molecules of air regardless of their chemical composition. This is a critical dynamic affecting the location of the tropopause. We should realize that there is no hard boundary between the ozone-sphere and the troposphere.
What accounts for the presence of absence of ozone? The atmosphere is opaque to solar radiation short of the visible wave lengths. Photolysis is the the splitting or decomposition of a chemical compound by means of light energy or photons in the short wave or ionizing spectrum. The presence of ozone is made possible by the splitting of the oxygen molecule by short wave ultraviolet. A few oxygen atoms recombine in the O3 form. As an even larger molecule than O2, ozone is more susceptible to photolysis than oxygen. Consequently the quantity of O3 in the stratosphere is not large, possibly as much as 10ppm which is one fortieth the concentration of that other greenhouse gas, carbon dioxide. The formation of ozone takes place at an elevation of 30km and above. At lower altitudes photolysis is progressively weaker because of the absorption of ionizing radiation at higher elevations . If it escapes the zone of active photolysis, ozone drifts downward but only to the extent that the movement of the air, and the antagonistic presence of water vapor will allow. In the equatorial region this down-drift is opposed by a moist updraft.
In the subtropics at 10-40° of latitude the down-drift of ozone is facilitated by the presence of dry air descending into high pressure cells in the troposphere. See figures 10 and 11 below and in particular figure 4 indicating a presence of appreciable ozone at altitudes that we consider to be within the ‘troposphere’. The presence of ozone in the ‘troposphere’ where there is ‘humidity’ and cloud cover influences that cloud cover and surface temperature.
In the polar regions there is an intermittent tendency for the air to descend, tending to produce a very broad minimum in surface temperature in the winter months. Polar air is usually warmer than the surface. Surface pressure is higher in winter encouraging descent. As the Antarctic Oscillation Index falls (an indicator of higher surface atmospheric pressure) the atmospheric column descends bringing ozone closer to the surface. Simultaneously, the night jet at the top of the stratosphere is invigorated bringing mesospheric nitrogen oxide into the upper stratosphere, eroding ozone and cooling the upper stratosphere. So, as surface pressure rises the upper stratosphere cools and the atmosphere below 50hPa warms all the way to the surface. Figure 2 charts the intermittent nature of that process. It is the change in surface pressure that determines the speed of the downdraft and the penetration of the night jet. Surface pressure changes on all time scales and it is itself changed by the process whereby the stratosphere and troposphere are coupled in convection.
Figure 2

Ozone as an agent for change and its distribution in low and mid latitudes
The presence of ozone in the upper troposphere/lower stratosphere is responsible for the inversion in temperature that begins at the tropopause. So, in the lower stratosphere (beneath the zone of active photolysis) we see the greenhouse effect in its most assertive manifestation and that effect is entirely due to the presence of ozone and its response to outgoing long wave radiation from the Earth.
Long wave radiation is a term used to describe the infrared energy emitted by the Earth (and its atmosphere) at wavelengths between about 4 μm and 25 μm (micrometers). Between 8 and 14 µm radiation passes readily through the troposphere. There is also a partial window for transmission in far infrared spectral lines between about 16 and 28 µm. Ozone absorbs round the 9.6 μm band. CO2 absorbs at a number of intervals in both shorter and longer wave lengths than ozone. A greenhouse gas that is stratified, such as ozone, markedly enhances the greenhouse effect because it absorbs at wave lengths that would not otherwise be absorbed and promotes emission at wave lengths that can be absorbed by complementary absorbers. Consequently, the greenhouse effect is much enhanced in the stratosphere by comparison with the troposphere.
But ozone is not particular to the stratosphere. It is found below the tropopause and above the stratopause. It is carried in and out of the tropospheric domain and as we will see, it does so most dramatically and influentially at high latitudes.
It can be observed that the stratosphere exhibits hot spots above deserts where radiation is enhanced. Locally, the temperature of the stratosphere (and the troposphere where it contains appreciable ozone) depends upon both ozone content and the amount of energy in transit.
In the troposphere, ascending air cools by decompression and it gives off little radiation. In locations where ascent is strong there is accordingly less radiation to heat the stratosphere. Secondly, in the tropics there is less ozone to absorb that radiation due to the strength of the updraft. This accounts for the coolness of the atmosphere at 100hPa through to 50hPa in the tropics. Note the deficiency in radiation in near equatorial latitudes between New Guinea and Pakistan and to the East of the Gulf of Mexico. This is the fingerprint of convection.
Figure 3
While ascending air cools by de-compression, descending air warms by compression and gives off abundant radiation. For this reason the high pressure cells of the subtropical latitudes (located somewhere between 10-40° of latitude depending upon season) are potent sources of long wave radiation emanating not from the surface but directly from the atmosphere. Since these cells are larger and most potent in the winter hemisphere we have the paradox of the Earth system delivering more radiation to space from its cooler hemisphere and very little radiation emanating from the tropics where the energy from the sun is most available.
Indeed the increase in radiation in winter produces a seasonal peak in the temperature of the stratosphere at 20-30°south latitude at a time when the surface is at its coolest. See figures 3 and 4. It is the presence of sufficient ozone in about a third of the upper troposphere that is responsible for this seasonal maximum in upper air temperature in the middle of winter. Of course, any change in the concentration of ozone in these latitudes will affect high altitude cirrus cloud. In the last post on this blog I documented the seasonal decline in relative humidity at 300hPa in the upper troposphere between the equator and 50° south in the middle of the year. That is a direct product of heating in the northern hemisphere because the strength of the downdraft in the southern hemisphere (and the temperature and humidity of the descending air) is related to the strength of the updraft in the north and the variable presence of ozone in the descending air in the south.What goes up must come down.
So, the notion that ‘the troposphere is heated from the surface’, while essentially valid, takes on a different twist when the upper troposphere contains ozone. The air temperature is then determined in exactly the same way as it is determined in the stratosphere. The implications for cloud cover should be plain. This is the essential feature of the natural climate change dynamic as it affects mid and low latitudes.
Figure 4 Temperature of the atmosphere at pressure levels 20-30° south
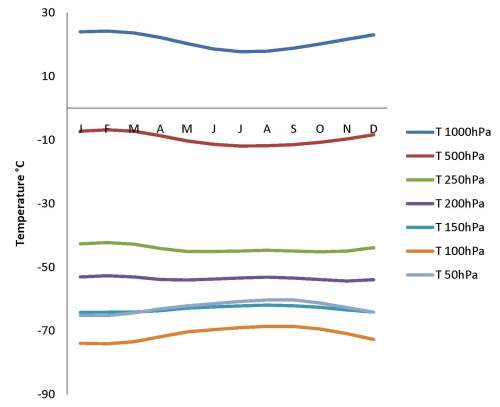
Source of data: http://www.esrl.noaa.gov/psd/cgi-bin/data/timeseries/timeseries1.pl
‘Convection’ is the term used to describe the displacement of less dense air by denser air. Any warming of the air will make it less dense. When water vapor condenses heat is released into the surrounding atmosphere. Again heat is released to the atmosphere as water turns to ice. Since water vapor is by and large confined to the troposphere, so too (except for a very important special case to be explored shortly) is convection. There is some overshooting of convection from the troposphere into the stratosphere in the tropics, especially over tropical rain forests where the supply of moisture to the atmosphere is more generous.
The fact that temperature falls with increasing altitude in the troposphere greatly assists the process of convection because a parcel of ascending air tends to be warmer than the air that surrounds it. Convection is a countervailing force to down-welling long wave radiation so far as any effect on the temperature of the surface is concerned. A noted already, rising air cools by decompression, not radiation. Any source of warmth expands the air and promotes convection. Convection is the defining characteristic of the troposphere. It has the effect of moving energy from low to high in the troposphere, from the summer to the winter hemisphere and at the surface displacing warm moist air polewards. The assertion that down-welling radiation from increased greenhouse gas will warm the surface flies in the face of our knowledge of the physical processes at work in the troposphere. It is a product of religion, not science. Hence the inappropriate demonization of carbon dioxide.
It is very clear from the map above that the southern hemisphere radiates strongly in winter. Were there a viable greenhouse effect, it would do a lot of good by warming the surface of the southern hemisphere in winter reducing the diurnal and annual range of temperature, especially over land. The annual range of temperature is indeed much truncated in the southern hemisphere, due to this effect (working to reduce cloud cover) and also the ratio of land to sea.
Does the stratosphere have water vapor? Clouds do occur in the stratosphere, especially in the tropics and subtropics where convection is strongest. Is water vapor in the stratosphere antagonistic to the existence of ozone? According to Greg Shindel at NASA “Water vapor breaks down in the stratosphere, releasing reactive hydrogen oxide molecules that destroy ozone. These molecules also react with chlorine containing gases, converting them into forms that destroy ozone. So a wetter stratosphere will have less ozone.” http://www.giss.nasa.gov/research/briefs/shindell_05/
Ozone in the polar atmosphere and the mobile ‘tropopause’
Can we speak of a ‘tropopause’ at high latitudes? The summer and winter situations are very different but in neither instance will we find a tropopause at 7 km in elevation as suggested by Wikipedia, not even an ‘indistinct’ one. Is there convection in the polar troposphere? Yes. There is no boundary to convection in the high latitude atmosphere. At high latitudes the atmosphere is heated in a top down rather than a bottom up fashion. It is at the top of the atmosphere that we find the agent of heating and convection, ozone in relative abundance. There is no heating to be had at the surface.
There is very little water vapor in the polar atmosphere to help us distinguish between ‘troposphere’ and ‘stratosphere’. Most of the moisture is squeezed out of tropical air in its transit from the equator. Accordingly the polar surface at high latitudes is a cold desert. Incidentally, it is just as well that the air is dry by the time it gets to the pole, otherwise more of the ocean would be permanently locked up in the polar ice cap. Does the extent of the polar ice cap (and sea level) primarily reflect the strength and humidity of the mid latitude westerlies? Is the extent of polar ice simply a function of the balance between accretion and depletion. But let me not be distracted.
Deprived of sunlight in winter one might imagine that the polar stratosphere might lose its ozone and become extremely cold. The reverse is the case. Photolysis by sunlight is the chief natural cause of ozone degradation. In a winter regime of zero photolysis, ozone accumulates in the polar stratosphere as is clearly apparent in figures 6 and 7. It is also apparent that ozone tends to be diminished in the low and mid latitudes of the winter hemisphere via the process of wasting into the high pressure cells of the mid latitudes in the winter hemisphere and this is clearly evident in the northern hemisphere.
Figure 6

Figure 7

Source: http://ds.data.jma.go.jp/gmd/jra/atlas/eng/atlas-tope.htm
The entire atmospheric column at the pole is below freezing point. On the face of it this, might be expected to produce a relatively still atmosphere. This is not the case. High latitudes experience destructive winds, especially in Antarctica, and on a more consistent basis than elsewhere. Strong wind is due to extreme pressure gradients. The manner in which extreme pressure gradients are created is unique to this part of the globe. The presence of ozone in the troposphere causes heating. But ozone is unequally distributed. The warmest parts of the stratosphere naturally ascend and the coolest parts descend into the domain of the ‘troposphere’, and in particular over the ocean. The presence of ozone causes a reduction in the number of molecules in the atmospheric column. Surface pressure falls. The lowest surface atmospheric pressure on the globe is to be found, not where the sun shines brightly, but at high latitudes where the sun is weak and the Earth itself is the source of radiation that heats the air. See figure 14 below.
In the upshot, as w see in figures 6 and 7 there is a very strong gradient in ozone concentration between the mid latitudes (low) and the Arctic and Antarctic circles (high) in the winter hemisphere. When the polar circulation forces ozone into the troposphere it makes for a dynamic situation with very strong gradients in surface pressure. This enhances wind strength in winter.
Figure 8
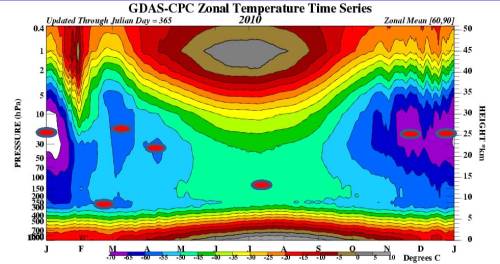
The process of convection at high latitudes is assisted by the upward movement of the ‘cold point’ in winter. In the Arctic summer in mid year the cold point lies somewhere between 8 and 20km in elevation (Figure 8). Yes, if this is the ‘tropopause’ it is manifestly ‘indistinct’. Actually, this thermal profile indicates that the process of convection that we associate with the troposphere would be readily extended into the stratosphere were the source of warmth to be associated with the surface. But, it is actually not associated with surface phenomena at all. It is associated with the presence of ozone. Accordingly the dynamic behind the degree of convection is the ozone content of the upper stratosphere.
Dynamics in the stratosphere
The night jet introduces nitrogen oxides from the mesosphere into the stratosphere between 1 and 50hPa, eroding ozone in summer and winter, with a bias towards stronger activity in the high pressure regime of winter.
In winter and spring the cold point moves up and down between 300hPa and 20hPa like a yo-yo. We see in figure 8 that the cold point can not be considered to mark the transition between a ‘troposphere’ and ‘stratosphere’. These terms have no application in the very different atmosphere at high latitudes.
In figures 9 and 10 we see that the temperature of the Arctic stratosphere at 5hPa gyrates in winter/spring in a spectacular fashion. In figure 9 the warming events are marked with red arrows (Arctic) and green arrows (Antarctic). Each warming event is associated with a decline in the temperature of the equatorial stratosphere at 5hPa. It is also associated with an increase in the AO as seen in 10. To see this connection please relate the timing of the warming episodes marked with green (Antarctic origin) and red (Arctic origin) lines in figure 9 to the timing of the increases in the AO as documented in figure 10. Since the AO relates to change in polar atmospheric pressure we can observe that every warming episode is due to a fall in polar surface pressure and a collapse in the night jet.
Figure 9
Source: http://www.cpc.ncep.noaa.gov/products/stratosphere/temperature/
It is apparent that the change in the temperature of the polar stratosphere has global implications for wind force and direction, including the Pacific Ocean where ENSO relates to a surface pressure oscillation. The notion (in UNIPCC type climate science) that heating of the upper stratosphere at the poles it is driven by ‘planetary waves’, ‘gravity waves’ or ‘heat flux from lower latitudes to high latitudes’ is nonsense. The heating of the atmospheric column is time specific, seasonal in occurrence, it relates to the presence or absence of NOx from the mesosphere and change in the surface pressure regime as represented in the AO and the AAO indices. Nowhere in science is there are more dramatic illustration of the impact of ideology in determining ‘orthodoxy’. The effect is to rule out of consideration the process by which the sun causes change in surface wind, air temperature and precipitation. The proponents of UNIPCC simply do not wish to know about this dynamic.
Figure 10

The global nature of this dynamic is apparent in the association of figures 9 and 10. We need to understand this if we wishe to understand how the climate can change change naturally. A rise in the AO (fall in atmospheric pressure at high latitudes) corresponds with the warming event in the upper polar stratosphere and a cooling event in the lower polar atmosphere. It is coincident with a marked cooling of the middle and upper stratosphere over the equator. As we will see it is also associated with an increase in the intensity of solar radiation reaching the ocean in mid latitudes. Simultaneously there is a reduction in atmospheric pressure at about 60° of latitude, an increase in pressure at about 30° of latitude and therefore a stimulation of the westerly winds.
Summarizing:
- Fall in the AO (rising polar pressure) = abrupt increase in the temperature of the lower polar atmosphere and simultaneous fall in the temperature of the upper polar atmosphere as the penetration of the night jet is enhanced. This is associated with an abrupt increase in the temperature of the equatorial upper stratosphere as the equator loses atmospheric mass, pressure falls there as it rises at the pole.
- Rise in the AO (falling polar pressure) = abrupt fall in the temperature of the lower polar atmosphere as the temperature of the upper polar stratosphere increases due to the night jet stalling. This is accompanied by a fall in the temperature of the upper equatorial stratosphere as the equatorial atmosphere gains mass. The increase in the temperature of the upper polar atmosphere is referred to as a sudden stratospheric warming. It is associated with enhanced ozone content. Such a warming is documented in late January 2011 in figure 8 and in August, September and October in figure 11.
These dynamics have been described as the Northern Annular mode and the Southern Annular mode of inter-annual climate variation. See http://www.atmos.colostate.edu/ao/introduction.html
Unique features that energize the Antarctic polar circulation
Figure 11

In the Antarctic the surface is colder than the Arctic all year round. The cold point is elevated for a longer period than it is in the Arctic. Looking at the lowest panel of figure 9 there is a weaker (than the Arctic) but persistent variation in the temperature of the upper atmosphere at 5hPa over nine months of the year. Only between February and April is this variation diminished. The persistence of the night jet over a longer period each year is associated with a diminished level of ozone in the southern stratosphere. That same relatively invariable persistence is also associated with a reduced incidence and intensity of ‘stratospheric warmings’.
The nature of the Antarctic atmosphere has changed dramatically over time.
Figure 12 The temperature of the stratosphere at 80-90°south in September. °C
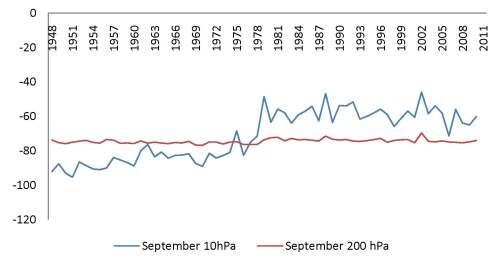
Since 1948 the temperature at 10hPa in September has increased strongly while that at 250hpa is stable. The increase in the temperature at 10hPa relative to 200hPa indicates enhanced thermal contrast in the upper ozone-sphere, favoring the overturning circulation. The increase in temperature aloft is consistent with the progressive decline in surface atmospheric pressure south of 50°south with compensating gain in pressure between 50° south and 50° north. This change in atmospheric pressure is documented in figure 14. It represents the unobserved gorilla in the climate change room. This gorilla is invisible in ‘climate science’ as it is propagated in the works of the UNIPCC.
Figure 14 Pressure Loss /Gain according to latitude between the first and last decades of the period 1948 -2011

The churning of ozone into the lower atmosphere produces the pattern of surface pressure that we see in figures 15 and 16. Notice the band of extremely low surface pressure on the margins of Antarctica and lesser zones in the North Atlantic and North Pacific.
Figure 15

Source: http://ds.data.jma.go.jp/gmd/jra/atlas/eng/atlas-tope.htm
The evolution of surface pressure in the northern hemisphere is plainly affected by the presence of the Eurasian land mass where temperature swings more wildly than it does over the sea. We see high pressure on the land in winter and low pressure in summer. In northern winter, low pressure anomalies (associated with ozone descent) affect latitudes between 50-60°north in particular over the North Pacific and North Atlantic. This is the location where the coupled circulation injects ozone into the lower atmosphere producing a tell tale pattern of sea surface temperature anomalies in mid latitudes.
In the southern hemisphere the coupled circulation produces a near continuous zone of low surface pressure on the margins of Antarctica that deepens in winter.
Figure 16

Source: http://ds.data.jma.go.jp/gmd/jra/atlas/eng/atlas-tope.htm
For an animation of global sea level pressure courtesy of Paul Vaughan see http://i54.tinypic.com/swg11c.png The animation shows the strong role of the Eurasian land mass in determining surface pressure in the northern hemisphere. Notice the seasonal increase in surface pressure over Antarctica in winter and the relatively invariable zone of low pressure on its margins.
The waxing and waning of surface pressure at 60-70° south is responsible for the variation in the strength of the westerly winds in the southern hemisphere that we see in Paul’s animation at: http://i52.tinypic.com/nlo3dw.png. Isotachs are lines of equal wind speed. Notice the increase in the strength of the westerlies in winter in the zone between South Africa and Australia consistent with the presence of the persistent ozone anomaly in this area, a feature that is likely connected with the phenomenon known as the Indian Ocean dipole.
Obviously there is a strong variability in surface pressure at 60-70° south (ozone churn zone) from month to month and across the year. That variation is due to changing ozone content of the polar stratosphere and change in the patterns of convection that are due to this variation. Once initiated the process of ozone churn actively lowers pressure between 50° of latitude and the pole. This reduces night jet activity allowing ozone content to further increase. In other words there is a powerful feedback mechanism. It is this feedback that has robbed the southern hemisphere of atmospheric mass at mid to high latitudes over the last sixty years.
The coupling of the stratosphere and the troposphere at the poles is continuous, albeit weaker in summer than winter. We know this from the pattern of geopotential heights (warmed air) produced in the upper troposphere. By charting the GPH anomalies we observe the effect of the coupled circulation at the poles on the upper troposphere at any time of the year.
Figure 16

Source: http://www.cpc.ncep.noaa.gov/products/intraseasonal/z200anim.shtml
The warming and drying influence of ozone on the upper troposphere, plainly affecting cloud cover and sea surface temperature, propagates towards the equator as we see below. Ozone is carried towards the equator by the counter westerly flow in the upper troposphere destroying ice cloud as it moves and allowing the ocean to warm. There are plainly many centers of activity in the southern hemisphere at 20-30° south. Relative stability in the geographical spread of these locations of enhanced ozone descent produces the pattern of global sea surface temperature anomalies that is characteristic of ENSO.
Figure 17 Association of geopotential height anomalies at 200hPa with sea surface temperature anomalies
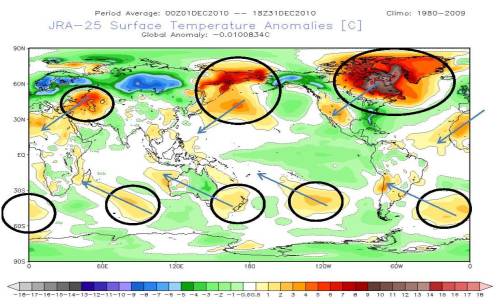
The warming of the sea in mid and low latitudes is directly associated with the fall in polar pressure on the margins of Antarctica. It is therefore associated with with an increase in the speed of the westerly winds and enhanced evaporation from the sea surface, a phenomenon particularly marked in the southern hemisphere. So, the sea surface temperature response in the southern hemisphere is muted by the evaporation response, a phenomenon much less evident in the northern hemisphere.
Figure 14, showing the loss of atmospheric pressure over time tells us that the stronger circulation is in Antarctica. The Arctic circulation is disrupted by heating of the northern land masses in summer. The relative lack of ozone churn in the Arctic stratosphere is reflected in higher stratospheric ozone values, weaker westerly winds and weaker polar easterlies. However this background of high ozone and weak wind strength is associated with strong changes in the domain occupied by the surface winds when quite small change in surface pressure occur. The change in pressure relations between mid and high latitudes has long been monitored as the Arctic Oscillation. When polar pressure is high the cold polar Easterlies sweep well south into former domain of the westerlies. When pressure is low the westerlies sweep further north and winters are much more benign. This is THE climate change dynamic that produces the swing in winter temperature in the northern hemisphere that repeats at regular intervals. 1960-78 cooling. 1978-2007 warming. Post 2007 cooling.
A brief note on the night jet
A picture is worth a thousand words.
1. As we have seen in relation to figure 9 and 10 the activity of the night jet varies with atmospheric pressure. See also: https://climatechange1.wordpress.com/2011/09/08/a-climate-change-dynamic/
2. It is known that the concentration of nitrogen oxides in mesospheric air depends upon solar activity.
3. There is a well documented relationship between geomagnetic activity and change in the surface pressure relationship between mid and high latitudes in winter (documented as the Arctic and the Antarctic Oscillation Indexes).
Consider now:
Figure 18

Source http://www.cpc.ncep.noaa.gov/products/stratosphere/strat_a_f/
Figure 18 shows the effect of the night jet on the ozone concentration of the upper stratosphere in August 2011 an elevation of roughly 43 km. In the core you see a zone of 5.5 to 6 ppm ozone. On the margins of Antarctica ozone attains 8.5 to 9 ppm and over the Pacific peaks at 11to 11.5 ppm ozone. The evolution of the night jet is the primary control on ozone content of the polar stratosphere. The extent of wasting of ozone into the troposphere is the secondary mode of control, and probably more influential, especially in the southern hemisphere. There may be other influences, perhaps related to the number of aerosol cans and fridges delivered to municipal rubbish tips, but in the big scheme these influences have been and continue to be inflated by hysteria, agenda driven politics and the demand for research funding by egocentric academics dedicated to a particular view of the world. That view of the world sees man as ‘the problem’.
Why is the coupled circulation over Antarctica so much stronger than it is over the Arctic? The southern hemisphere lacks the mass of land that exists in the northern hemisphere to warm the atmosphere in summer and disrupt the polar circulation.
Summary
The polar circulation injects ozone into the high latitude lower atmosphere. With little water vapor the atmosphere at high latitudes possess few of the properties of the low or mid latitude troposphere. It is this perturbation in ozone content affecting the weight of the atmospheric column (and surface pressure) that changes the wind, the cloud and surface temperature on the inter-annual, decadal and centennial time scales. It does this by changing the concentration of the one greenhouse gas that is beyond the influence of man. The presence of absence of ozone in the cloud zone is a matter for the sun and the atmosphere in a complex dance that challenges our imagination. It is the electromagnetic influence of the sun, depending upon plasma density within the neutral atmosphere that initiates a redistribution of the atmosphere and starts a process that includes a strong feedback mechanism. The feedback mechanism is in turn dependent on the night jet and surface pressure. It is the activity of the sun that determines the chemical constitution of mesosphere air that is drawn into the tenuous upper atmosphere over the poles. It is the sun that ultimately controls this circulation. The Earth system greatly amplifies the tiny stimulus that the sun applies to the tenuous upper atmosphere.
In this way the cloud comes and goes. Global cloud and high altitude ice cloud in the southern hemisphere is most abundant between November and March when the Arctic circulation is influential. It is at this time when the Earth is closest to the sun that the most vigorous variation in the El Nino Southern Oscillation is seen. If we look deeper, we see a strong dependency of the Arctic circulation on the Antarctic. On the shortest time scale the Arctic circulation is frequently a mirror image of that in the Antarctic. On the longest time scale the AO moves with the AAO. The AAO sees by far the largest swings on decadal and longer time scales. The Antarctic is not only the strongest circulation, it is the least studied.
One can restate this argument in a slightly different way. An ozone feed to the troposphere from either pole changes high altitude ice cloud density. Observation reveals that surface temperature varies most widely between November and March when the Arctic circulation is most active. See https://climatechange1.wordpress.com/2011/10/03/where-is-science/ It is at this time we see the greatest fluctuations in the Southern Oscillation index that reflects pressure variation between Tahiti and Darwin.The Southern Oscillation Index leads all sea surface temperature indices. Surface pressure change has its origin in change in the AO and the AAO.
What is described here is a natural climate change dynamic that represents the primary mode of climate variation affecting the Earth system. To the extent that temperature in the tropics is aligned with change in surface pressure (it is) you know that this factor is influential. The primacy of the Antarctic in terms of its influence on surface pressure indicates that the Antarctic is ‘The Main Player’ with the Arctic acting as the necessary intermediary in mediating the process of change.
The oft repeated proposition that change is initiated at low latitudes and propagates towards the poles is precisely 180° out of whack. When we speak of the influence of ENSO on global temperature we are actually looking at the product of polar processes.
Note: For a detailed analysis download the document at http://www.happs.com.au/images/stories/PDFarticles/TheCommonSenseOfClimateChange.pdf
Earlier posts on this topic can be accessed at https://climatechange1.wordpress.com/2011/01/17/earths-changing-atmosphere/ and earlier at https://climatechange1.wordpress.com/2009/11/08/the-climate-engine/